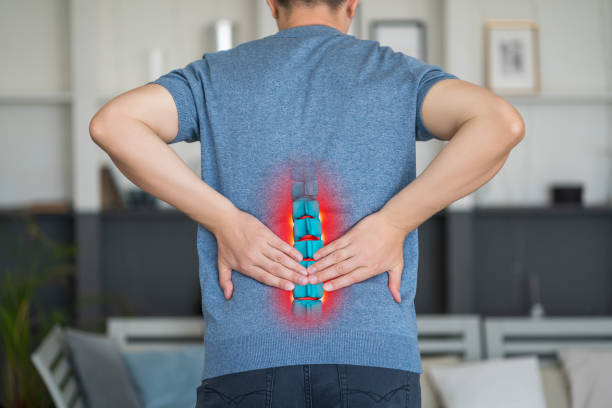
Understanding Metabolic Acidosis
Metabolic acidosis is a condition characterized by an accumulation of acids in the body, which disrupts the normal pH balance. This section provides an overview of metabolic acidosis, its causes, symptoms, as well as the diagnosis and treatment options available.
Overview of Metabolic Acidosis
Metabolic acidosis occurs when there is an excess of acid production or a reduced ability of the kidneys to eliminate acids from the body. This imbalance in acid-base regulation leads to a decrease in blood pH below the normal range of 7.35 to 7.45.
There are several underlying causes of metabolic acidosis, including:
- Untreated diabetes: When diabetes is not properly managed, the body cannot effectively use glucose for energy. This can result in the production of acidic ketones, leading to metabolic acidosis.
- Loss of bicarbonate: Bicarbonate is an important buffer that helps maintain the acid-base balance in the body. Conditions such as severe diarrhea or renal tubular acidosis can cause excessive loss of bicarbonate, contributing to metabolic acidosis.
- Kidney conditions: Chronic kidney disease or kidney failure can impair the kidneys' ability to excrete acids, leading to the accumulation of acids in the body and the development of metabolic acidosis.
Causes and Symptoms of Metabolic Acidosis
The symptoms of metabolic acidosis can vary depending on the underlying cause and the severity of the condition. Common signs and symptoms may include:
- Accelerated heartbeat
- Confusion or cognitive impairment
- Fatigue or weakness
- Shortness of breath
- Nausea or vomiting
- Headache
Severe cases of metabolic acidosis can lead to life-threatening complications, including shock and organ dysfunction [1].
Diagnosis and Treatment of Metabolic Acidosis
Diagnosing metabolic acidosis involves a thorough evaluation of medical history, physical examination, and laboratory tests. Blood tests, such as arterial blood gas analysis, help measure the pH level, bicarbonate levels, and other parameters to confirm the presence of metabolic acidosis. Urine tests may also be conducted to assess kidney function and determine the cause of the acidosis [1].
Treatment of metabolic acidosis focuses on addressing the underlying cause and restoring the acid-base balance. The specific treatment approach will depend on the severity and underlying condition. Some treatment options may include:
- Medications: Sodium bicarbonate or other alkalizing agents may be prescribed to help neutralize acids and restore the pH balance in the body.
- Dietary changes: Modifying the diet to reduce acid-producing foods and increase the consumption of alkaline-rich foods can help manage metabolic acidosis.
- Treatment of the underlying condition: Addressing the root cause of metabolic acidosis, such as managing diabetes or kidney disease, is essential for long-term management.
In severe cases, when metabolic acidosis is life-threatening, hospitalization and intravenous administration of fluids and medications may be necessary to stabilize the condition.
Understanding the causes, recognizing the symptoms, and seeking timely medical intervention can help manage metabolic acidosis effectively. It is important to work closely with healthcare professionals to determine the most appropriate treatment plan based on individual needs and circumstances.
The Myth of LSD and the Spinal Cord
There is a common misconception that LSD (lysergic acid diethylamide) can remain entangled in the spinal cord. However, this idea is not supported by scientific evidence. LSD is rapidly metabolized by the liver and eliminated from the body within a matter of hours [2]. Let's explore the metabolism of LSD, the duration of its effects, and its mechanism of action.
Metabolism of LSD in the Body
After ingestion, LSD is quickly metabolized by enzymes in the liver. The primary metabolite of LSD is 2-oxo-3-hydroxy LSD (O-H-LSD), which is further metabolized into other compounds that are eventually eliminated from the body through urine. This rapid metabolism contributes to the relatively short duration of the drug's effects.
Duration of LSD Effects
The effects of LSD typically last for 6 to 15 hours after ingestion. However, the exact duration can vary depending on factors such as the dose taken, individual metabolism, and environmental conditions. It's important to note that while the primary effects of LSD may subside within this timeframe, residual effects, such as changes in perception and mood, can persist for a longer period.
Mechanism of Action of LSD
LSD exerts its effects by primarily interacting with serotonin receptors in the brain. Specifically, it binds to the serotonin 5-HT2A receptors, leading to altered perceptions, thoughts, and emotions. This interaction with the serotonin receptors is thought to modulate the release and activity of neurotransmitters, resulting in the characteristic hallucinogenic effects of LSD.
It's important to note that LSD is not physically addictive, but it can lead to psychological dependence and tolerance with regular use. The exact mechanisms underlying these psychological effects are still being studied, and individual experiences can vary.
In summary, the idea that LSD remains in the spinal cord is a myth. LSD is rapidly metabolized by the liver and eliminated from the body within hours. Its effects, which are primarily due to interactions with serotonin receptors in the brain, typically last for 6 to 15 hours. Understanding the true metabolism, duration, and mechanism of action of LSD can help dispel misconceptions surrounding its effects on the spinal cord.
Spinal Cord Injuries: Causes and Consequences
Understanding spinal cord injuries is crucial in comprehending the potential consequences and the impact they can have on an individual's life. This section provides an overview of spinal cord injuries, the different types and their symptoms, as well as the diagnostic testing involved.
Overview of Spinal Cord Injuries
Spinal cord injuries (SCI) are a debilitating condition that can result from various causes, such as traumatic accidents, falls, sports injuries, or medical conditions. These injuries occur when there is damage to the spinal cord, leading to a disruption in the normal functioning of the nerves within the spinal canal.
The consequences of a spinal cord injury can be severe and life-altering. They can vary depending on the location and severity of the injury. In some cases, spinal cord injuries can cause paralysis, loss of sensation, muscle weakness, and other impairments. The impact on an individual's physical abilities, mobility, and overall quality of life can be significant.
Types and Symptoms of Spinal Cord Injuries
Spinal cord injuries can be classified into two main types: complete and incomplete. A complete injury refers to a total loss of motor and sensory function below the level of the injury. This means there is no voluntary movement or conscious sensation below the affected area. In contrast, an incomplete injury implies that there is some preservation of motor function and sensation below the level of injury.
The symptoms experienced with a spinal cord injury can vary depending on the extent and location of the damage. Common symptoms include loss of movement, loss of sensation, muscle weakness or paralysis, difficulty breathing, changes in sexual function, and bladder or bowel dysfunction. The severity of the symptoms can also differ, ranging from mild impairment to complete loss of function.
Diagnostic Testing for Spinal Cord Injuries
When a spinal cord injury is suspected, prompt and accurate diagnosis is crucial for appropriate medical management. Diagnostic testing plays a vital role in assessing the extent and location of the injury.
Computed tomography (CT) scans are often used as an initial diagnostic tool to evaluate spinal cord injuries. CT scans can provide detailed images of the spine and surrounding structures, allowing healthcare professionals to identify any fractures, dislocations, or other abnormalities. Additionally, magnetic resonance imaging (MRI) may be utilized to further assess the spinal cord, nerve roots, and soft tissues, providing more comprehensive information.
Diagnostic testing for spinal cord injuries may include the following:
- Computed Tomography (CT) scans - including CT brain without contrast, CT cervical spine without contrast, and CT chest, abdomen, and pelvis with maximum intensity protocol (MIP) with and without contrast.
- Magnetic Resonance Imaging (MRI) - which can provide detailed images of the spinal cord and surrounding structures, aiding in the assessment of the injury.
Accurate and thorough diagnostic testing is essential for determining the appropriate treatment plan and guiding rehabilitation efforts for individuals with spinal cord injuries.
Understanding the causes, types, and symptoms of spinal cord injuries, as well as utilizing advanced diagnostic testing, is crucial in providing timely and effective medical care for individuals affected by these injuries. By gaining a deeper understanding of spinal cord injuries, healthcare professionals can work towards improving outcomes and enhancing the quality of life for those living with these challenging conditions.
Debunking the LSD Flashback Myth
There is a common myth surrounding LSD, suggesting that it remains in the spinal cord and can cause flashbacks years after use. However, this belief is not supported by scientific evidence. LSD, or lysergic acid diethylamide, does not stay in the spinal cord or any other tissues for extended periods. Instead, it is swiftly metabolized by the liver and eliminated from the body within a matter of hours.
Persistence of LSD in the Body
LSD has a short half-life, meaning it is rapidly broken down by the body. This short duration of action is due to the drug's metabolism and elimination processes. Consequently, the idea that LSD persists in the spinal cord or other tissues for an extended period is not substantiated by scientific research.
Psychological Triggers of Flashbacks
Flashbacks, often referred to as "acid flashbacks," are more likely to be triggered by psychological associations rather than the physical presence of LSD in the spinal cord or other tissues. These associations can be influenced by various factors, including memories, emotions, and environmental cues. It is important to understand that flashbacks are subjective experiences and do not indicate the continued presence of LSD in the body.
LSD and Urinary Excretion
LSD is known to be one of the fastest-drugs to be eliminated from the body due to its water solubility. It is rapidly excreted through urine. This quick elimination further supports the notion that LSD does not remain in the spinal cord or body for an extended duration.
In conclusion, the belief that LSD stays in the spinal cord and causes flashbacks years after use is a myth. Scientific research and evidence indicate that LSD is swiftly metabolized and eliminated from the body, making it unlikely to persist in the spinal cord or other tissues. Flashbacks, if experienced, are more likely to be triggered by psychological factors rather than the physical presence of LSD.
Advancements in Spinal Cord Injury Research
Despite the challenges that come with treating spinal cord injuries (SCI), advancements in medical research have paved the way for potential breakthroughs in regenerative therapy. Scientists and researchers are actively exploring various strategies to promote nerve repair and restore function in individuals with SCI. This section will delve into some of the challenges faced in treating SCI, as well as the cell-based and molecule-based strategies that show promise in this field.
Challenges in Treating Spinal Cord Injuries
Spinal cord injuries pose significant challenges for medical professionals due to the limited regenerative capacity of the adult central nervous system. The pathophysiology of SCI involves a combination of primary trauma and secondary injury, resulting in the loss of function and motor control. The breakdown of the blood-spinal cord barrier, infiltration of immune cells, activation of astrocytes, and the formation of glial scarring contribute to the cascade of damage and hinder regeneration.
Prevention of SCI is crucial since many causes are avoidable. Road traffic accidents and falls from heights are among the leading causes of SCI globally. By addressing the risk factors associated with these accidents, the incidence of SCI can be significantly reduced.
Cell-Based and Molecule-Based Strategies
Regenerative therapy for chronic spinal cord injuries involves two primary approaches: cell-based and molecule-based strategies. These innovative methods aim to promote nerve repair, axonal growth, and mitigate glial scarring.
Cell-Based Therapies: This approach involves transplanting specific types of cells into the damaged spinal cord to promote regeneration. Stem cells, neural precursor cells, and olfactory ensheathing cells are among the cell types being studied for their potential in restoring function and connectivity in the injured spinal cord. Research in this area is ongoing, and although some progress has been made, further studies are needed to optimize the effectiveness of cell-based therapies.
Molecule-Based Strategies: Molecule-based strategies focus on targeting inhibitory molecules in the injured spinal cord to encourage axonal growth, degrade glial scarring, and stimulate the intrinsic regenerative capacity of the spinal cord. One promising molecule under investigation is acidic fibroblast growth factor (aFGF). Studies have shown that aFGF promotes axonal growth and mitigates glial scarring, offering potential for nerve repair in SCI.
Acidic Fibroblast Growth Factor for Nerve Repair
Acidic fibroblast growth factor (aFGF) has emerged as a potential therapeutic agent for nerve repair in spinal cord injuries. Over the past two decades, multiple trials using aFGF have been conducted in both human and animal experiments. These studies have shown promising results, demonstrating the potential of aFGF in promoting axonal growth and mitigating glial scarring in nerve injuries.
The use of aFGF in regenerative therapy for spinal cord injuries represents an exciting avenue of research. However, further studies and clinical trials are necessary to fully understand its efficacy and safety in human patients.
As research continues to advance, the hope for more effective treatments and potential recovery options for individuals with spinal cord injuries remains strong. By exploring various strategies and approaches, scientists and medical professionals strive to improve the lives of those affected by SCI and enhance their chances of regaining function and independence.
The Role of Metabolism in the Body
Metabolism is a complex process that plays a crucial role in the functioning of the human body. It involves a series of chemical reactions that convert food into energy, allowing the body to perform various functions. Understanding the role of metabolism is essential in comprehending how the body processes substances and maintains equilibrium. This section will explore three key aspects of metabolism: anabolism and catabolism, basal metabolic rate (BMR), and influential factors on metabolic rate.
Anabolism and Catabolism
Metabolism can be divided into two interconnected processes: anabolism and catabolism. Anabolism, also known as constructive metabolism, focuses on building and storing, supporting new cell growth, body tissue maintenance, and energy storage. During anabolism, the body utilizes energy to synthesize complex molecules, such as proteins and carbohydrates, from simpler substances. This process is essential for growth, repair, and the overall maintenance of the body.
On the other hand, catabolism, also referred to as destructive metabolism, involves the breakdown of large molecules, such as carbohydrates and fats, to release energy. Through catabolic processes, the body breaks down these complex substances into simpler forms, such as glucose, which can be readily utilized by cells to generate energy. Catabolism provides the necessary fuel for various cellular activities and bodily functions.
Basal Metabolic Rate (BMR)
Basal metabolic rate (BMR) is a measure of how many calories a person's body burns while at rest. It represents the energy required to maintain essential bodily functions, such as breathing, circulating blood, and regulating body temperature. BMR is influenced by various factors, including genetics, body composition, and exercise. Individuals with more muscle and less fat generally have higher BMRs. Muscles require more energy to maintain than fat, contributing to a higher metabolic rate.
BMR can vary among individuals, and it can be affected by age, gender, and hormonal factors. For instance, the hormone thyroxine, produced by the thyroid gland, plays a significant role in determining the speed and direction of metabolic chemical reactions in the body, influencing the overall metabolic rate.
Influential Factors on Metabolic Rate
Several factors can influence an individual's metabolic rate. These include:
- Body composition: The proportion of muscle mass and fat in the body can impact metabolic rate. Muscle tissue requires more energy to maintain, leading to a higher metabolic rate compared to fat tissue.
- Physical activity: Regular exercise and physical activity can increase metabolic rate. Engaging in aerobic exercises, strength training, and other forms of physical activity can help boost metabolism even after the activity has ended.
- Genetics: Genetic factors can influence metabolic rate. Some individuals may have a naturally higher or lower metabolic rate due to inherited traits.
- Hormonal factors: Hormones, such as thyroxine and insulin, play a role in regulating metabolic rate. Imbalances in these hormones can affect how efficiently the body processes and utilizes energy.
- Caloric intake: The number of calories consumed through food and beverages directly affects metabolic rate. Consuming more calories than the body needs can lead to weight gain, while consuming fewer calories can cause the body to conserve energy and potentially lower metabolic rate.
Understanding the role of metabolism and the factors that influence it is essential for maintaining a healthy body weight and overall well-being. By adopting a balanced diet, engaging in regular physical activity, and considering individual metabolic differences, individuals can optimize their metabolic rate and support their overall health.
Maintaining Acid-Base Balance in the Body
Acid-base balance is essential for maintaining homeostasis in the human body. The pH of the body, which measures the acidity or alkalinity, plays a crucial role in various biological processes, including the oxygenation of blood and the functioning of biochemical reactions. The normal pH range in the human body is 7.35 to 7.45, with an average of 7.40.
Importance of Acid-Base Balance
Maintaining the acid-base balance is vital for the overall health and proper functioning of the body. Deviations from the normal pH range can have significant implications. If the pH becomes too acidic (acidemia) or too alkaline (alkalemia), it can disrupt enzyme activity, affect the delivery of oxygen to tissues, and impact cellular function. Therefore, the body has built-in compensatory mechanisms to counterbalance disturbances in pH levels.
Compensatory Mechanisms in pH Regulation
When the body experiences a disturbance in pH levels, it activates compensatory mechanisms to restore the balance. For instance, if a person develops metabolic acidemia (increased acid levels in the blood), the body will try to compensate by inducing a respiratory alkalosis. This compensatory response involves increased breathing rate (hyperventilation), leading to a decrease in carbon dioxide levels and an increase in pH. However, it's important to note that compensation may not completely normalize the pH, and the terminology is used to distinguish between individual processes and the overall pH status of the patient.
Role of Carbon Dioxide and Buffer Systems
Carbon dioxide (CO2) plays a crucial role in maintaining acid-base balance in the body. It is produced as a byproduct of aerobic cellular respiration and combines with water to form carbonic acid (H2CO3), a weak acid. This reaction occurs naturally but is facilitated by the enzyme carbonic anhydrase, which assists in the conversion of CO2 to H2CO3. Carbonic acid can then dissociate into bicarbonate ions (HCO3-) and hydrogen ions (H+), contributing to the pH regulation in the body.
Buffer systems in the body, such as the bicarbonate buffer system, phosphate buffer system, proteins, and hemoglobin, also play a crucial role in maintaining pH balance. These buffer systems act as chemical reservoirs, absorbing excess hydrogen ions or releasing them to maintain a stable pH. They help prevent large swings in pH levels and contribute to the overall acid-base balance in the body [7].
Understanding the mechanisms involved in pH regulation is essential for maintaining optimal health and preventing disturbances in the acid-base balance. The renal system and the pulmonary system are the two main modulators of pH balance in the body. The pulmonary system adjusts pH using carbon dioxide, while the renal system reabsorbs bicarbonate and excretes fixed acids to regulate pH. The pulmonary system can compensate for metabolic pH disturbances within minutes to hours, while the renal system's compensation takes longer, usually days.
Arterial blood gas (ABG) sampling is a diagnostic test used to assess the acid-base status of a patient. It measures parameters such as pH, partial pressure of carbon dioxide (pCO2), bicarbonate (HCO3-), and oxygen saturation. ABGs are particularly important in adjusting the management of critically ill patients, especially those requiring ventilator support.
By understanding the importance of acid-base balance, compensatory mechanisms, and the role of carbon dioxide and buffer systems, we can appreciate the complexity of pH regulation in the body and the significance of maintaining a stable acid-base environment for optimal physiological functioning.
The Renal and Pulmonary Systems in pH Regulation
The body maintains pH balance through the interplay of various systems, with the renal and pulmonary systems playing vital roles in regulating pH levels. Let's explore how these two systems contribute to maintaining acid-base balance.
pH Regulation by the Renal System
The renal system, which includes the kidneys, plays a crucial role in pH regulation. It accomplishes this through the reabsorption of bicarbonate ions and the excretion of fixed acids. Bicarbonate ions act as a buffer to maintain pH balance in the body. The kidneys selectively filter blood, reabsorbing bicarbonate ions and eliminating hydrogen ions and ammonium ions to eliminate excess acid from the body.
This process involves the filtration of blood in the kidneys, followed by the reabsorption of bicarbonate ions. The kidneys also excrete hydrogen ions and ammonium ions to regulate the elimination of acid. By adjusting these processes, the renal system helps maintain the acid-base equilibrium in the body.
pH Regulation by the Pulmonary System
The pulmonary system, which includes the lungs, also plays a significant role in pH regulation. It achieves this by adjusting the levels of carbon dioxide in the body. When carbon dioxide levels increase, such as during metabolic acidosis, the pulmonary system responds by increasing ventilation. This increased ventilation helps remove excess carbon dioxide from the body, restoring pH balance.
By adjusting the respiratory rate and depth, the pulmonary system can effectively regulate the elimination of carbon dioxide. This process helps maintain the acid-base equilibrium in the body.
Acid-Base Balance and Arterial Blood Gas Sampling
To assess the acid-base status of a patient, healthcare professionals may perform arterial blood gas (ABG) sampling. This test measures parameters such as pH, partial pressure of carbon dioxide (pCO2), bicarbonate (HCO3), and oxygen saturation. ABGs are particularly important in adjusting to the needs of critically ill patients and guide ventilator management.
Arterial blood gas sampling provides valuable information about a patient's acid-base balance, allowing healthcare providers to evaluate the effectiveness of pH regulation by the renal and pulmonary systems. By analyzing the levels of pH, pCO2, and HCO3, healthcare professionals can make informed decisions about treatment and interventions.
The renal and pulmonary systems work together to maintain pH balance in the body. While the pulmonary system can compensate for metabolic pH disturbances within minutes to hours, the renal system's compensation takes longer, usually days. This collaborative effort ensures that the body's acid-base balance is regulated and maintained, enabling optimal physiological functioning.
References
- [1]: https://my.clevelandclinic.org/health/diseases/
- [2]: https://firststepbh.com/blog/does-acid-stay-in-your-spine/
- [3]: https://www.ncbi.nlm.nih.gov/pmc/articles/PMC8710452/
- [4]: https://action-rehab.com/does-lsd-stay-in-your-spine/
- [5]: https://www.ncbi.nlm.nih.gov/pmc/articles/PMC6944993/
- [6]: https://kidshealth.org/en/teens/metabolism.html
- [7]: https://www.ncbi.nlm.nih.gov/books/NBK507807/